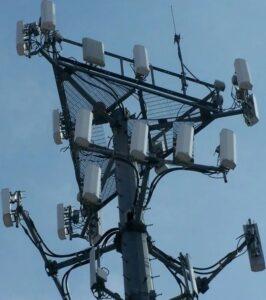
Comprehensive understanding of MIMO
In order to give full play to the potential of 5g Gbps data transmission speed and ultra-low delay, mobile operators need to improve the performance of all network parameters. This means a lot of investment in spectrum acquisition, network infrastructure and transmission technology. No matter how it is implemented, the cost of deploying 5g nationwide is very high for mobile network operators. Providing 5g services at a lower cost is the biggest obstacle to popularizing 5g technology. Although high-frequency millimeter-wave has attracted much attention, operators still use sub-6ghz large-scale MIMO technology to minimize costs and deploy 5g in the national mobile network.
MIMO (multiple input multiple output) is an antenna technology of wireless communication. It uses multiple antennas to send and receive signals.
Unlike traditional wireless communication, which usually uses a single antenna, MIMO sends the same data in the form of multiple signals through different antennas. In this way, spatial multiplexing can be realized, in which each channel transmits independent information to the receiver. Therefore, compared with the traditional single antenna, MIMO has many advantages.
When the RF signal encounters obstacles such as buildings, the signal will scatter and reach the target receiver through different paths. In a single antenna system, this multipath propagation will lead to poor reception, call disconnection and sharp decline in data transmission speed. MIMO radio can receive transmission streams that combine multiple identical data, so multipath propagation can be used to improve signal quality and strength. If the scattering in the propagation environment is rich enough, many independent subchannels will be generated in the same allocated bandwidth, so as to achieve quality and signal gain without additional bandwidth or power. Network operators can focus on building more antennas to meet demand, rather than more base stations.
MIMO antenna array can also focus the signal in a single user direction by using beamforming and beam control technology. A single antenna transmits wireless signals in all directions, while through digital and analog methods, multiple antennas can focus signals in specific directions and point to the receiver, which greatly improves spectral efficiency and power efficiency.
5G large scale MIMO
Wireless technologies of all ages have used the progress of MIMO antenna array technology to improve the network speed. 3G introduces single-user MIMO, which uses multiple synchronous data streams to transmit data from the base station to a single user. 4G system uses multi-user MIMO technology to allocate different data streams to different users to achieve obvious capacity and performance advantages. Using 5g new wireless standard, MIMO can realize “large-scale” deployment. 4G system is usually equipped with four transmit antennas and four receiving antennas, namely 4×4 antenna array. 5g large-scale MIMO adopts more transmitting and receiving antennas to improve transmission gain and spectral efficiency; Some arrays reach 256×256.
Because large-scale MIMO uses more antennas, the signal beam sent to the receiver is much narrower. In this way, the base station can provide RF energy to customers more accurately and effectively. The phase and gain of each antenna are controlled separately, and the channel information will be retained in the base station, so the mobile device does not need to use multiple receiver antennas. A large number of base station antennas will improve the signal-to-noise ratio of the base station, so as to improve the capacity and throughput of the base station.
It is also important that 5g technology is based on 4G network infrastructure and can share spectrum with previous technologies through dynamic spectrum sharing. In this way, mobile network operators can improve network capacity, support high-speed data transmission, save spectrum and reduce operating costs as much as possible.
The future of millimeter-wave, sub-6 GHz reality
Millimeter-wave technology (or mmwave) and 5g are often mistaken for synonyms. Millimeter wave is a frequency band in the 24GHz to 100GHz RF spectrum used by 5g network, and sub-6 GHz refers to the “low-frequency band” and “below 6 GHz” frequencies. Because the signal propagation loss of this frequency band is high and will be blocked by buildings, leaves, rain and human body, we previously thought that millimeter wave was not suitable for mobile communication. However, these short wavelengths can transmit more data over a short distance. Obviously, in order to achieve the target of 5g 20GB / s data rate, millimeter wave spectrum needs to be used finally. Although many people in the field of mobile communication are excited about its development prospects, the logistics challenges faced by promoting this technology across the country have not been paid enough attention.
This is particularly obvious if the millimeter wave is viewed from the perspective of the base station. The transmission range of millimeter wave base station is smaller than that of base station tower transmitting lower frequency signals. Researchers estimate that in order to achieve national coverage, U.S. network operators will need to build 13 million base stations. In contrast, the number of base station towers supporting today’s mobile networks in the United States is about 300000. Since the cost of meeting the millimeter-wave power consumption requirements is very high, the capital expenditure for realizing these millimeter-wave base stations nationwide is further increased. In addition to stadiums and urban hotspots, it is unrealistic to deploy millimeter wave technology across the country in the next few years.
Although OEM manufacturers are trying to reduce the cost of millimeter wave technology, 5g network operators will still rely on the sub-6 GHz band. Low frequency signals can further penetrate obstacles such as buildings and cover a larger area around the base station tower before disappearing, so they are suitable for rural and urban areas. This means that the sub-6 GHz 5g network can achieve greater coverage through fewer base stations, and can use the existing base stations of the operator.
Large scale MIMO infrastructure requirements
Although the 5g network of sub-6 GHz cannot achieve a significant speed increase like millimeter wave technology, its large-scale MIMO antenna array can support more synchronous connections, improve signal throughput, and achieve the best balance between user coverage and capacity. Therefore, 5g of sub-6 GHz is a more practical way to realize. Compared with millimeter wave deployment, 5g of sub-6 GHz can more quickly improve the speed and consistency of mobile broadband. While moving towards a fully integrated 5g network, it can also improve the current 4G system in real time. Therefore, many people in the industry hope that operators will bid for a lower spectrum range in order to provide 3G, 4G and 5g services in the same frequency band by using dynamic spectrum sharing. We have seen international 5g implementation schemes using this method. South Korea launched low-frequency 5g two years ago, and China will reform its entire network infrastructure to achieve nationwide 5g coverage in the next few years.
This is not to say that the 5g deployment of sub-6 GHz is very simple; These new technologies will also bring great system design challenges. In order to adopt large-scale MIMO technology on 5g base station, designers need to develop a highly complex system containing hundreds of antenna elements. Many systems use active phase array antennas to provide dynamic beamforming and beam control functions to specific users. All these additional antennas can improve performance, but these large antenna arrays require more power and require the use of dedicated RF front end (RFFE) chipsets and amplifiers.
Building an RF front end to support these new sub-6 GHz 5g applications will be a challenge. RFFE circuit is very important to the power output, selectivity and power consumption of 4G system. 5g modulation mechanism puts forward additional requirements, so the wireless infrastructure power amplifier (PA) needs to have very high efficiency to achieve the required linearity. In addition, the huge difference between peak power and minimum power requirements will bring heat dissipation problems to the power amplifier and RF front end.
报错 笔记